Developing Hydrogels for Bioprinting Astrocytes
A Promising Step Towards 3D Brain Tissue Models
Investigating the human brain
Understanding the human brain remains one of the most prominent branches in science. While scientists have made significant progress, further advancements are hindered due to the lack of effective human brain models. Often, researchers rely on complex 2D co-culture methods or animal models. These can be both expensive and time-consuming, and do not accurately represent the human brain.
Dr. Anna Herland at KTH Royal Institute of Technology and Dr. Daniel Aili from Linköping University recognized the need to enable researchers to develop more biomemitic models of sophisticated brain-like structures such as the brain-blood-barrier (BBB) and the central nervous system. The BBB plays a crucial role in filtering compounds in the bloodstream. It ensures the brain tissues are supplied with nutrients, hormones and smaller molecules while being protected from pathogens and harmful particles.
The team has always been fascinated by how cells are affected by different materials and how they interact with each other in the microenvironment and recognized the importance of mimicking the extracellular matrix (ECM) environment to develop accurate 3D models of the BBB. To achieve this, culturing astrocytes in 3D was a critical step.
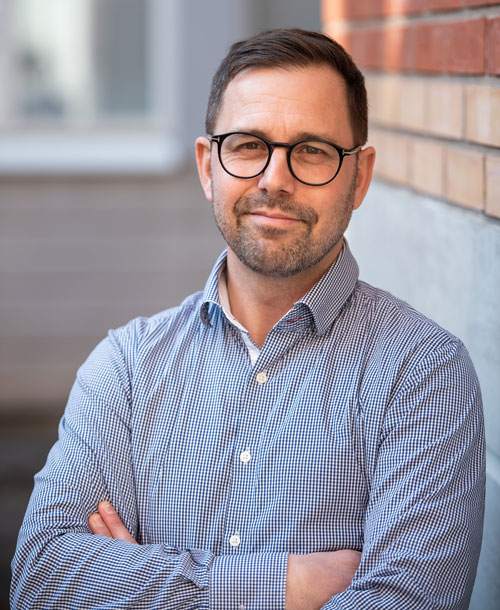
Photographer: Olov Planthaber, Linköping University
The role of astrocytes and the hydrogel they are cultured in
Astrocytes are the most abundant cell type in the brain and have numerous functions. Astrocytes provide structural support to neurons, regulating the levels of chemicals in the extracellular space, and help to form the BBB. They also play a role in the repair and regeneration of damaged neural tissue, and in the modulation of synaptic activity, which is important for learning and memory.
For the researchers, it was important to understand how astrocytes are affected by the materials they are cultured in. The close collaboration between the team of Dr. Anna Herland and Dr. Daniel Aili led them to develop a modular and bioprintable hydrogel system that could mimic key aspects of the ECM and would allow for tuning interactions between fetal primary astrocytes and the hyaluronan (HA) based hydrogels.
The hydrogels contained peptide cell adhesion motifs, cRGD and IKVAV. These motifs were chosen as the have been shown to improve cellular attachment in synthetic ECM models. Furthermore, they increase the ability of cells to differentiate into neurons and promote growth of branches.
Engineering hydrogels for astrocytes with bioprinting
The objective of the team was to create a hydrogel for neuron-like cells, specifically astrocytes, that is suitable for advanced biofabrication methods such as bioprinting. This would enable the creation of more intricate 3D models with precise structures and control over the composition and distribution of hydrogel and cells. One of the driving forces to develop engineered hydrogels as opposed to biologically derived hydrogel matrices, was to enable more reproducible experiments. Synthetic hydrogels allow for better control over the behaviour of cells and ensures consistency of the bioink.
To ensure the possibility to use an astrocyte-laden hydrogel as a bioink for bioprinting, Dr. Aili leveraged the BIO X and a syringe printhead. The team printed structures in a grid pattern and analyzed the cell number and morphology.
After optimizing the hydrogel system, the team confirmed it prints with good resolution despite being a softer biomaterial. It also remained stable and supported cell growth. The team observed high cell viability during and after the printing process and that the stress incurred by the cells was minimal.
“The printing process was very straightforward. The only factor we were not sure about was how the astrocytes will tolerate the bioprinting process. We saw a large difference based on what hydrogel composition we used. But with the right hydrogel composition, the cells did great.”
A key step toward replicating the complexity of structures in the brain
During a 6-day experiment, it was observed that printing astrocytes using the HA-based hydrogels enabled the cells to spread out their branches and connect to nearby cells in a pattern that forms a network. The specially designed hydrogels enabled the astrocytes to be printed, grown, and maintained. This breakthrough creates the potential to start replicating the intricate composition and spatial complexity of structures in the brain. Which is crucial for developing more advanced brain tissue and disease models.
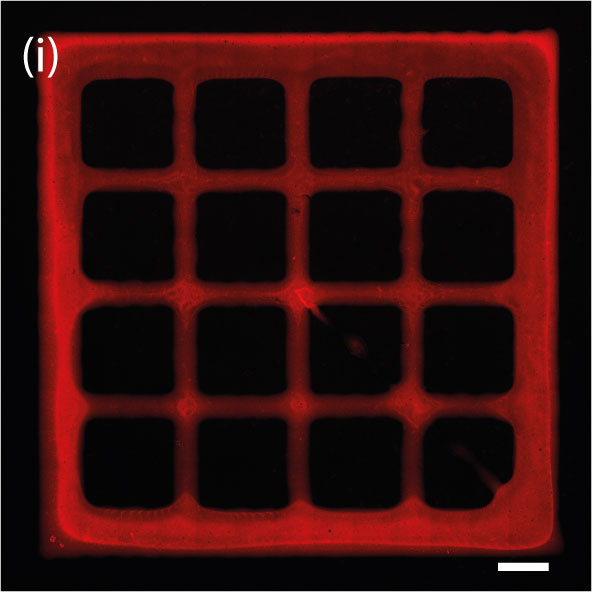
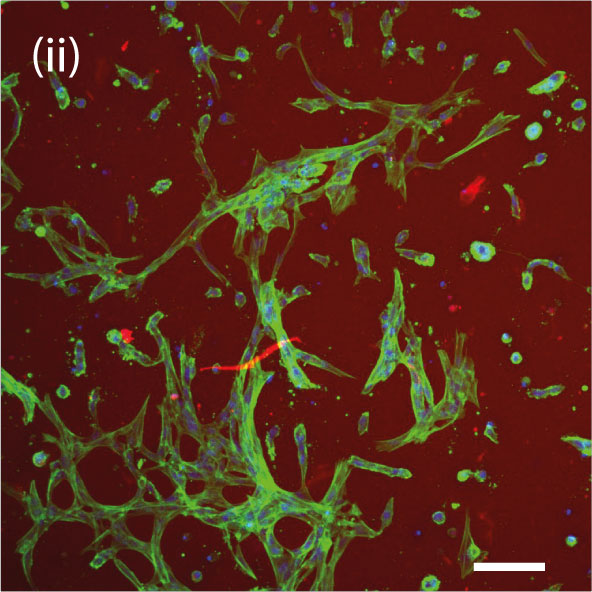
Applications in neuroscience
The group’s work has promising applications in neuroscience. 3D models of the BBB can answer fundamental questions. For example, in drug development 3D models would provide a tool to study and better understand how drugs can permeate the BBB.
The team plans to continue collaborating to develop models for both healthy and disease tissues, and also work closely with clinicians. Input and participation from clinicians are key to success and to ensure the research questions being asked are relevant.
Bioprinting creates the opportunity to study more fascinating questions
For nearly 5 years, Dr. Aili’s research group has been utilizing the BIO X bioprinter. According to Dr. Aili, having access to the bioprinter aligns well with his group’s research focus.
"The access to the bioprinter has allowed us to ask more interesting research questions, and has enabled us to be able to do our current research."
Any advice?
We had the opportunity to ask Dr. Aili for advice to scientists who would like to integrate bioprinting into their research. He encouraged researchers to take the leap and try bioprinting. However, he also emphasized the importance of having a deep understanding of both the materials and cellular systems involved.
Dr. Aili suggested that bringing together a diverse group of individuals with varying backgrounds and knowledge is crucial in this field.