Advancing lung cancer research
Creating a perfusable 3D model with DLP bioprinting
Lung cancer remains a major global health concern, with rising incidence and mortality rates.
Despite the rise in clinical treatment tools, numerous patients have limited chances to utilize drugs with higher success rates than chemotherapy. The urgency to discover new medications with improved effectiveness, capable of extending survival and combating drug resistance, has driven efforts to identify potential targets and advance promising therapeutic alternatives.
Navigating drug development challenges in lung cancer
Current research often relies on 2D cell cultures and animal models, but they fall short in replicating the complexity of in vivo tumors and human physiology. This results in a high failure rate in the process of translating drugs for clinical use. Over 92% of drug candidates that undergo testing in animals do not prove effective for human treatments, and this rate increases to a staggering 97% for anticancer drug candidates during clinical trials.
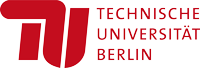
Recognizing the pressing need, Prof. Jens Kurreck and his team at Technische Universität Berlin, acknowledged the importance of identifying alternative methods for screening and testing new drugs for lung cancer. To facilitate the discovery of new effective drugs, the team turned to bioprinting to develop a human cancer model that would enable the study of drug effectiveness in a context that closely resembles real-world conditions.
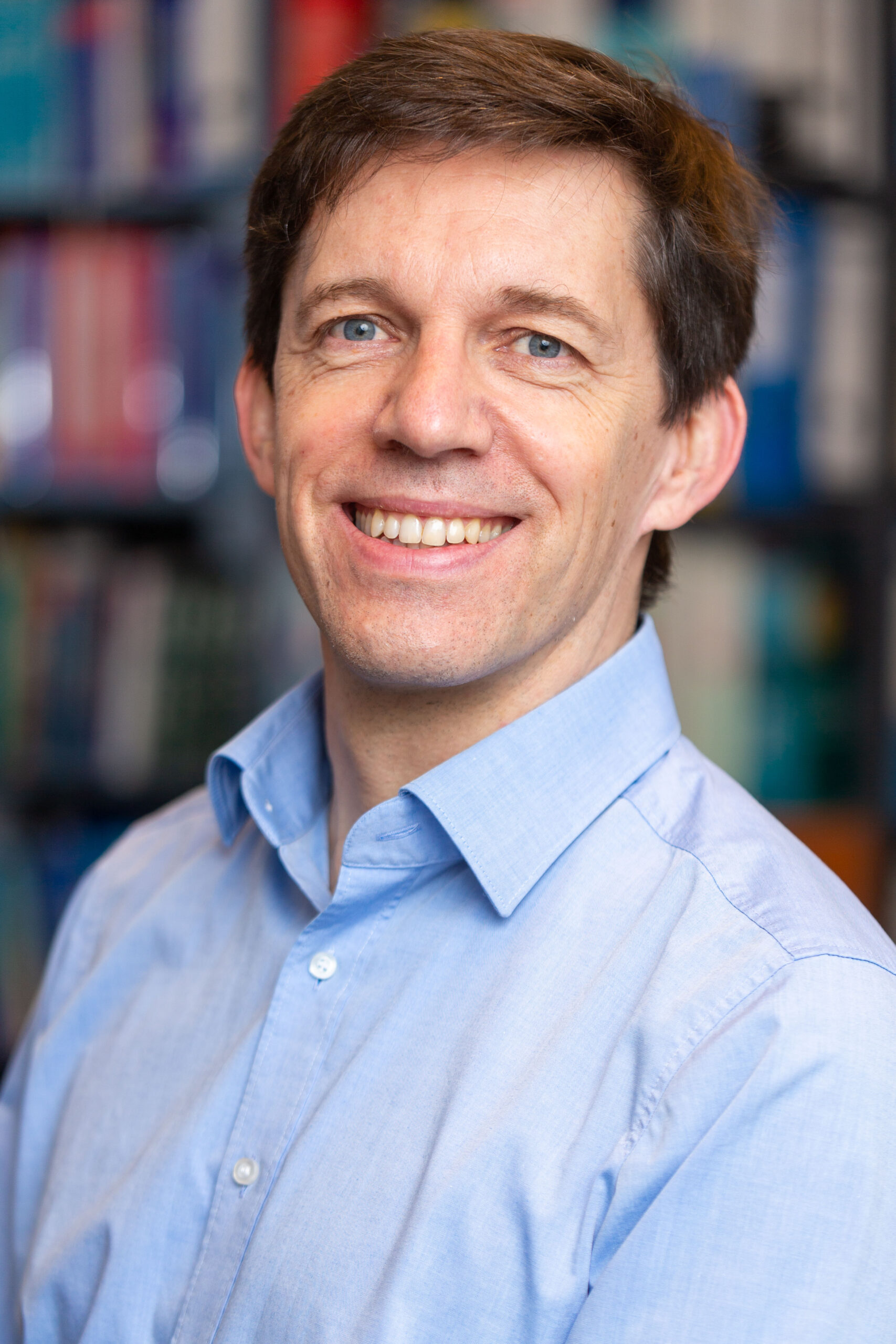
“There is a huge difference in causes of diseases and their underlying mechanisms between animal models and humans. This is why we believe it is important to have human organ models and bioprinting enables us to produce these models with high accuracy.”
– Prof. Jens Kurreck
3D bioprinting enables the creation of highly physiologically relevant 3D models with customizable designs, the incorporation of various human cells, and the adjustment of extracellular environment stiffness. A key benefit of bioprinting in the context of this project is the ability to include channels that simulate vascular structures like blood vessels.
Vascularization: mimicking natural tissue physiology
“Our goal was to fabricate an easily adoptable lung cancer model with a central vascular channel.”
Fabricating a perfusable 3D lung cancer model
While the team considered extrusion bioprinting, they ultimately chose to use CELLINK’s LUMEN X digital light processing (DLP) bioprinter for their research project. This decision was influenced by the bioprinter’s high-resolution capabilities and the effective chemical crosslinking of GelMA, which allowed the team to create vascular structures that were both highly precise and exceptionally stable.
The team used a bioink composed of methacrylated gelatin (GelMA) and the NSCLC (non-small cell lung cancer) cell line H358 to produce the first lung cancer model by DLP bioprinting. Post printing, the model showcased stability with the high toughness. This is in line with human tumors, which usually have high tissue stiffness. Moreover, the model was configured with an inlet and an outlet, enabling the establishment of a perfusable system that facilitated the delivery of nutrients and oxygen through a peristaltic pump. This setup enabled a comparison between 3D models cultured under static and dynamic conditions.
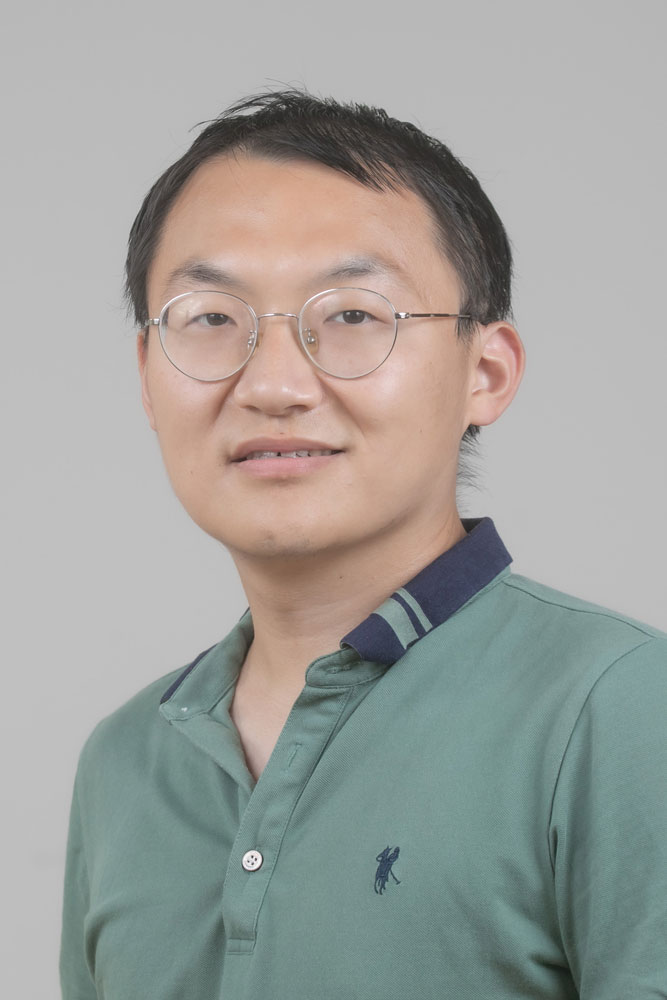
Copyright: Christian Kielmann
Yikun Mei, a member of Prof. Kurreck’s team, added:
“Furthermore, it’s worth mentioning that perfusion has the most straightforward impact on cell models by significantly enhancing the model’s cell viability. After all, perfusion provides a continuous supply of nutrients and oxygen. Additionally, compared to extrusion-based printing, DLP printing has simplified 3D printing considerably. You don’t need to fine-tune various printing parameters, repeatedly test and adjust your printing inks, or maintain calcium ion concentrations in the culture medium to sustain the model’s condition after printing.”
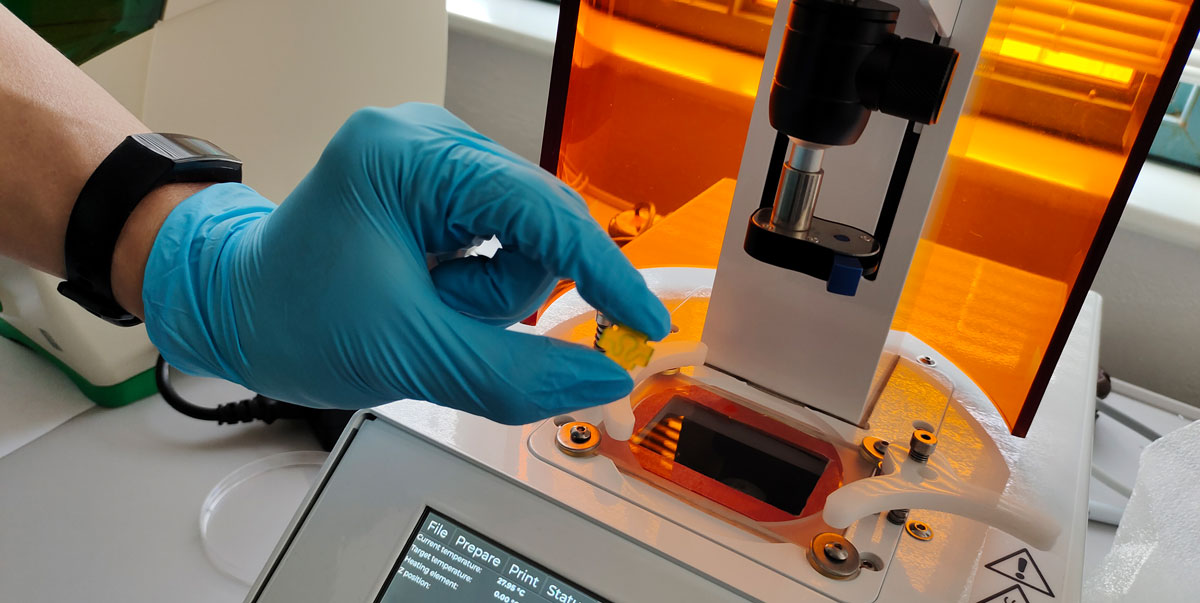
Redefining drug testing with 3D bioprinted models
The team investigated the efficacy of the cytostatic drug gemcitabine in different conditions, including in 2D cell cultures, controlled 3D models without perfusion (static) and 3D models with perfusion (dynamic). The control models were cultured under static conditions with the drug in the surrounding medium. On the other hand, the anticancer drug was delivered through the media flow in the perfused model, mimicking the process of drug delivery through the bloodstream in a human patient.
The experiments showed that higher concentrations (about 1000 times higher) were required to induce cell death in the static 3D cell culture, compared to 2D cell culture. In fact, the drug did not kill all cells even at the highest concentrations used. This difference is attributed to the dense network created by the hydrogel and cells in 3D cultures, mirroring the conditions of dense tumor tissue in patients. The density often poses challenges for the penetration of anticancer drugs into cancer tissues.
Prof. Kurreck explains:
“If you have a monolayer culture, all cells are in direct contact with the drug, so you need a lower concentration of the drug. In contrast, in the biological organ or tumor, things are arranged in three-dimensional space and then the drug must diffuse through the ECM. It must pass by other cells and that is why you need higher concentrations of the drugs.”
Drug testing under dynamic conditions
Conducting the experiment with perfusion in the 3D model under dynamic conditions clearly illustrated two significant findings: firstly, that perfusion enhances cell viability during extended cultivation, and secondly, that the drug’s efficacy is improved in this setup compared to the 3D static model. These results showcase the significance of incorporating a vasculature system within the 3D model.
Overall, the reduced cytostatic impact observed in 3D culture under dynamic conditions more closely resembles the biological scenario seen in human patients compared to the high efficacy of the cancer drug observed in 2D cultures.
3D cancer lung model for widespread reproducibility
The model developed by the team can be readily adopted by other teams operating in the realm of lung cancer research, seeking to make models more accessible, as Prof. Kurreck explained to us:
“In the existing literature there are some excellent models. However, they were created using exclusive printers, making it difficult for others to access and replicate the research. Our goal is to transfer knowledge and make it accessible to a broad audience.”
Advancing drug testing for clinical translation
“Ultimately, the key is to determine which approach, human-based models, or animal-based models, offers superior predictive capabilities. We believe that 3D models based on the human system, while not identical to whole organisms, possess significant predictive potential for human patients, especially in the context of organ and cancer models. By continuing our collaborative efforts and advancing human systems, we can achieve a higher level of translatability to benefit human patients.”
The team’s goal is to get to a point where they will start testing drug libraries in a perfused bioprinted organ model to be able to select new drugs. In addition, they want to combine various cell types, which has been a limitation in DLP bioprinting, until the recent release of the Advanced Mode on the BIONOVA X. Another important step, which can be enabled with multi-material DLP printing, is to distinguish whether a drug candidate has a high specific toxicity to the cancer cells, but not the healthy cells.
Partnership with CELLINK
The TU Berlin team acquired their first bioprinter in 2016 and has since expanded their collection to include a comprehensive range of bioprinters, including the INKREDIBLE+, BIO X, BIO X6, and LUMEN X models. They have maintained a close collaborative relationship with CELLINK during their projects.
Embracing bioprinting and the power of collaboration
“It is always good to get in contact with someone who is experienced in bioprinting. For example, we collaborated with another group from Austria and helped them to gain firsthand experience and insights into the practical aspects of bioprinting.“
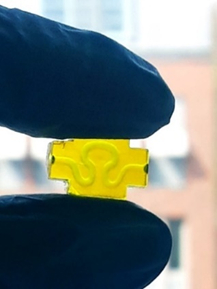