Microfluidics, Explained
The intersection of microfluidics and 3D bioprinting marks an exciting frontier in the field of biological technologies. As the dynamics of these two domains intertwine, new possibilities for advancements in biomedical research, drug discovery, and personalized medicine emerge.
What is Microfluidics?
Microfluidics is a technology that controls fluids at the microscale, providing precision and efficiency for cellular and molecular assays within microfluidic devices. It minimizes costs and time and sparks scientific discoveries.
The technology has been adapted into “microfluidic devices”, which are essentially miniaturized labs etched onto a single chip. These devices typically contain a series of tiny channels, chambers, and valves, through which fluids can be manipulated with high precision. Here, the complexity and breadth of biological assays that could be performed are only limited by the design of the chip.
One of the most innovative applications of microfluidic devices is in the development of “organ-on-a-chip” technology. An organ-on-a-chip (OOC) is a multi-channel 3-dimensional microfluidic cell culture chip that simulates the activities, mechanics and physiological response of entire organs and organ systems. This is a type of artificial organ that merges tissue engineering with microfluidics. These organs-on-a-chip can be utilized in various scientific and medical fields, offering an alternative to traditional in-vitro methods and animal testing. The integration of microfluidic devices and organs-on-a-chip is a prime example of how technology is advancing biological research.
How are microfluidic chips created?
Microfluidic chips are an integral part of the microfluidic landscape. Their creation involves a series of complex processes, marked by precision and adherence to stringent design principles. The production process can be broadly divided into three main stages: design, fabrication, and testing..
1. Design of the device
The first stage involves the design of the chip. Here, the chip layout is created using computer-aided design (CAD) software. The design process is guided by the specific requirements of the application the chip is intended for. This may include factors such as the desired fluid flow, heat exchange, and chemical reactions.
2. Fabrication of microfluidic devices
The production of microfluidic devices, often termed as ‘organs on a chip’, can be achieved through various fabrication methods. The four most common techniques are 3D bioprinting, soft lithography, photolithography and injection molding. Each comes with its own characteristics, advantages and disadvantages, which we delve deeper into in the table below.
Method | Characteristics | Equipment Needed | Advantages | Disadvantages |
3D Bioprinting | Allows for the creation of custom complex structures using biological materials and cells. | 3D Bioprinter and biomaterials | It can create intricate and personalized designs, allows for the incorporation of cells and different biomaterials, and has the potential for constructing functional tissues. | Necessitates thorough fine-tuning of printing parameters and involves a substantial investment in equipment, biomaterials, and cells. |
Soft Lithography | Relies on the molding and casting of elastomeric materials. | Mold, elastomeric material, curing agents | Basic and swift process, low cost, capable of producing flexible devices. | Limited to relatively simple designs, potential issues with material compatibility and durability. |
Photolithography | Employs light to transfer a geometric pattern to a photosensitive material. | Light source from UV to NIR (~365 nm to 780nm), mask, photosensitive material | Can achieve high resolution and precise patterns, widespread use in microfabrication. | Potential issues with material compatibility, complex process if etching and layers are needed. |
Injection Molding | Uses a mold into which a material is injected and solidified to create a final product. | Injection mold, material to be molded | Efficient for mass production, consistent quality, wide range of material choices. | High initial cost for mold design and fabrication, not ideal for low volume production. |
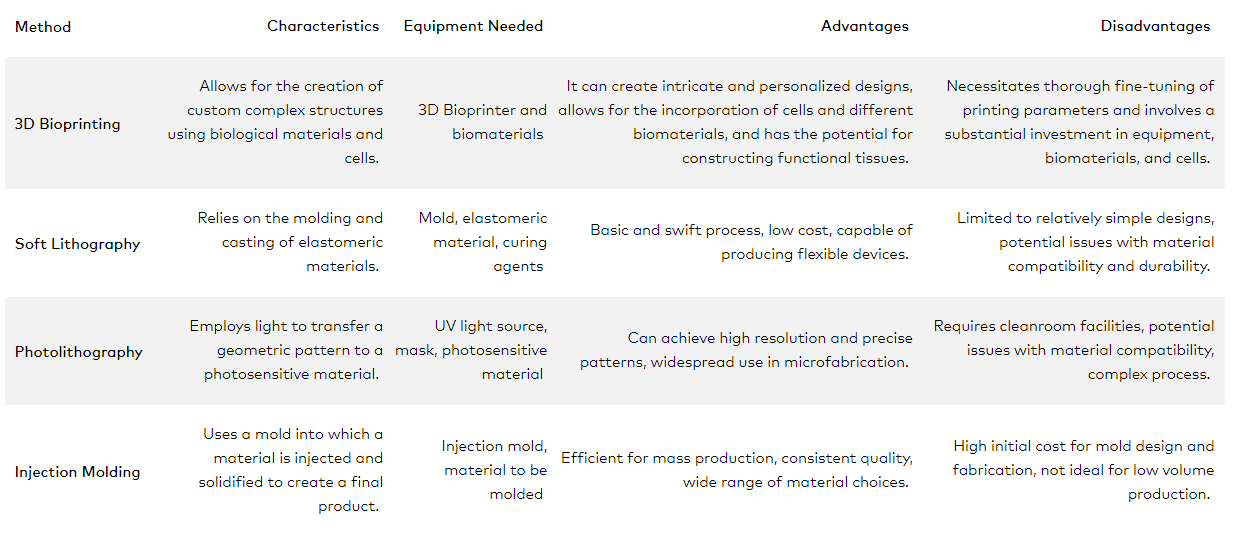
3. Testing microfluidic integrity
The final stage in the creation of microfluidic chips is testing. This involves verifying that the fabricated chip meets the design specifications. Several techniques can be used to test different aspects of the chip’s functionality. For instance, optical microscopy can be employed to check for structural integrity, while flow visualization techniques can be used to assess fluid dynamics within the microchannels.
Failure to produce microfluidic chips that meet design specifications can lead to a range of negative consequences. This underscores the critical importance of rigorous testing in the development process.
- Flawed Results: Incorrect chip design can cause inaccurate experimental results.
- Damaged Samples: Deviation from the design can damage or contaminate biological samples.
- Waste of Resources: Unreliable chips lead to wasted resources and increased research time.
Given these potential repercussions, the significance of testing cannot be overstated. It ensures that the performance of the chip aligns with the expected standards, enhancing the reliability of the device. Testing is a critical step that validates the functionality and accuracy of the microfluidic device, thereby contributing to the success of downstream applications.
Note: Proper testing methodologies are not just an optional step, but an integral part of the microfluidic chip creation process that ensures the quality, accuracy and reliability of the end product.
3D Bioprinting of Microfluidic Chips
3D Bioprinting is an emerging method for creating microfluidic chips. This process extends the capabilities of traditional 3D printing by using bio-inks made of living cell suspensions, extracellular matrices, and hydrogels. The technology allows for the precise structuring of living tissues layer-by-layer, which is particularly beneficial in the field of microfluidics.
In the creation of microfluidic chips, 3D bioprinting can be used to construct chips with complex geometries and multiple layers. These chips can then be tailored to mimic the physical and biochemical environments of human tissues or organs. This capability is especially useful in the development of organ-on-chip applications, which are devices that can simulate the physiological response of entire organs.
Moreover, 3D bioprinting can incorporate a variety of cell types into the microfluidic chip, enabling the creation of highly specific and personalized models. For instance, it’s possible to print patient-specific cells into the chip, providing a powerful tool for personalized medicine and drug testing.
LUMEN X
CELLINK’s LUMEN X is a prime example of how 3D bioprinting is redefining the capabilities of microfluidics. Using Digital Light Processing (DLP) technology, the LUMEN X bioprinter can create highly precise microfluidic chips with unparalleled speed. The device leverages biocompatible light-sensitive resin, allowing for the generation of complex, multi-layer structures with exceptional resolution.
The LUMEN X stands out in the realm of organ-on-chip application development. Its advanced bioprinting capabilities enable the construction of accurate, patient-specific organ models. By incorporating a patient’s own cells into the microfluidic chip, LUMEN X allows for a highly personalized approach to drug testing and disease modeling, bringing us one step closer to the era of personalized medicine.
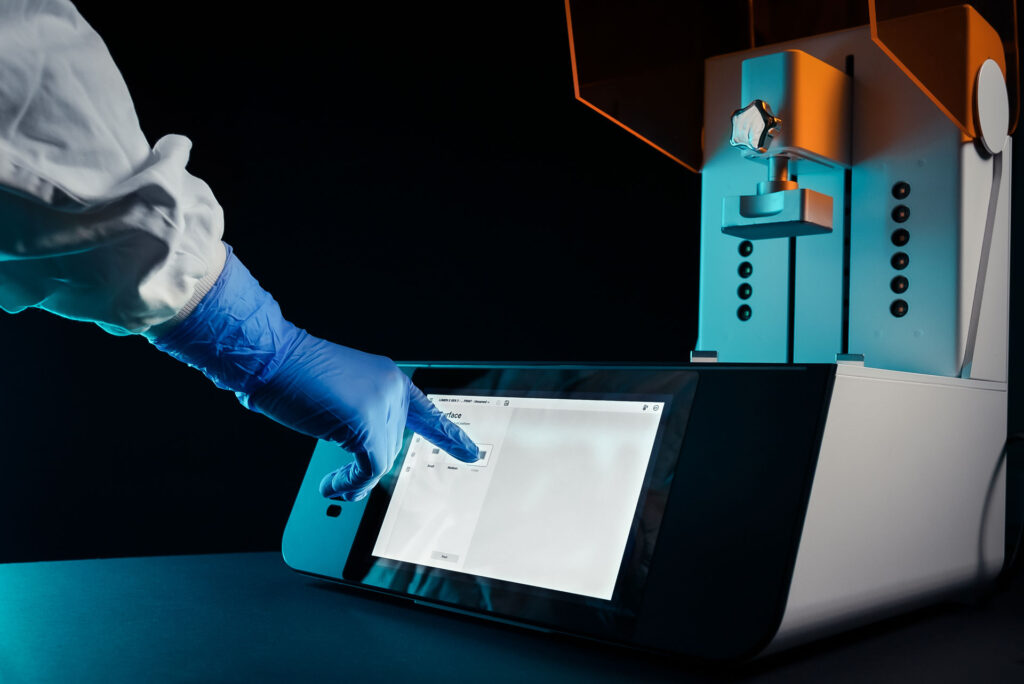
Applications of Microfluidics
Microfluidics is rapidly gaining traction in life sciences, proving to be a revolutionary technology with a wide array of applications, among them cell biology, drug discovery, point of care diagnostics, and tissue engineering.
Cell Biology and Analysis
Microfluidic devices are ideal for cell biology research, providing precise control over cellular environments. They enable the study of cell behavior under tightly controlled conditions, helping to reveal fundamental biological processes. For example, microfluidics can be used to study cell migration, proliferation, and differentiation, all critical aspects of development and disease processes. However, a promising alternative to traditional microfluidic systems is emerging: the 3D bioprinting technology, which combines the principles of 3D printing with biological sciences; it is a groundbreaking technology that can biofabricate structures that closely mimic in vivo tissues.
The structures created with this technology can be used for various purposes, including drug testing, disease modeling, and potentially organ transplants, thus providing a superior alternative within the biology sphere.
Drug Discovery and Development
In the pharmaceutical industry, microfluidics is enhancing drug discovery and testing. Micro-scale devices allow for high-throughput screening of drug candidates, accelerating the discovery process. Furthermore, the precision of microfluidics supports highly accurate drug dosage and delivery studies, facilitating the development of more effective and safer drugs.
Microfluidics technology is crucial for understanding a drug’s impact on multiple organ systems simultaneously (often called a body-on-a-chip system, or BOC system). Organ-on-a-chip models replicate human physiology in labs, with interconnected chambers housing cells from different organs. These models mimic complex interactions between organ systems, providing accurate insights into drug effects. The microchannels enable drug circulation, allowing real-time observation of organ responses and dynamic drug behavior. Organ-on-a-chip models also aid in studying drug toxicity and identifying potential side effects early in the drug discovery process. They offer a more realistic physiological environment, enhancing predictability in clinical trials and expediting drug development.
Point-of-Care Diagnostics
Microfluidic devices’ compact size and automation capabilities make them ideal for point-of-care diagnostics. These devices can quickly and efficiently analyze small samples, such as a drop of blood or saliva, and deliver rapid results. This has significant implications for disease diagnosis, particularly in remote or resource-limited settings. Adding to their appeal, microfluidic devices can be fashioned to perform multiple tests concurrently, thereby multiplying their diagnostic potential. They can assess multiple biomarkers in a single sample, enabling comprehensive disease profiling. This, in turn, allows for personalized diagnosis and treatment plans, reflecting the growing trend towards precision medicine in the healthcare sector.
Importantly, the use of microfluidic devices in point-of-care diagnostics can eliminate or significantly reduce the need for complex laboratory equipment and specialized personnel. This democratizes access to state-of-the-art diagnostic tools, and by extension, quality healthcare. Moreover, it expedites the timeline from sample collection to diagnostic result, a crucial factor in managing time-sensitive conditions.
Tissue Engineering & Regenerative Medicine
Printed with the Quantum X. Photo courtesy of Nanoscribe.
Finally, microfluidics is playing a transformative role in tissue engineering and regenerative medicine. Microfluidic organ-on-a-chip devices enable the creation of accurate models of human tissues and organs, critical for studying disease development and testing potential treatments.
A standout feature of CELLINK’s LUMEN X is its capability to create vascularized models. In the realm of tissue engineering and organ-on-chip technology, the ability to incorporate vasculature – the network of blood vessels – brings a significant leap toward mimicking the intricate complexity of human tissues and organs. With a vascularized model, we can replicate not only the structure but also the function of living tissues, leading to enhanced insights.
Vascularization is crucial for delivering nutrients and oxygen to cells, removing waste products, and facilitating cellular communication – all essential parameters for maintaining cell function and viability. LUMEN X’s precision in bioprinting enables the creation of such intricate networks, presenting an opportunity to study diseases in more realistic models and test therapeutic interventions in conditions that closely mirror the in vivo environment.
In essence, the LUMEN X does not just build models; it brings them closer to life. The result is a powerful tool in the advancement of bioengineering, medical research, and the development of personalized therapeutic strategies. The incorporation of vascularized models is a game-changer, elevating the complexity and the potential for deeper insights and breakthroughs.
CELLINK's Microfluidic Solutions
As the leading experts in the 3D bioprinting field, CELLINK is here to enable and help with the fabrication of your microfluidic devices and organ-on-a-chip systems. Book a demo to get a more thorough understanding of the vast capabilities of our 3D bioprinters.
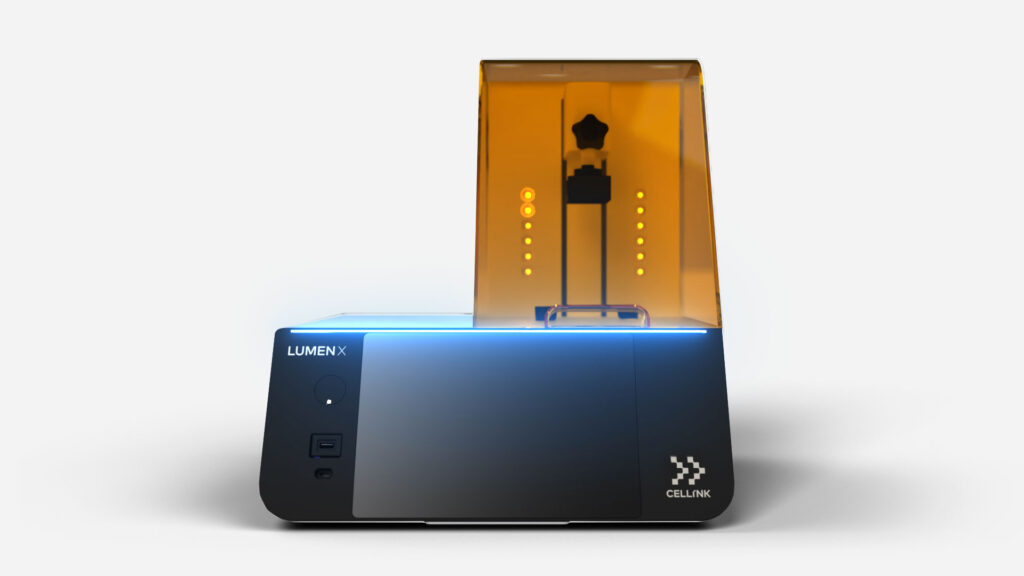
LUMEN X
With 35 μm pixel resolution, this DLP bioprinter enables the creation of intricate structures that closely mimic the natural cell environments, allowing for the creation of realistic organ-on-a-chip devices.
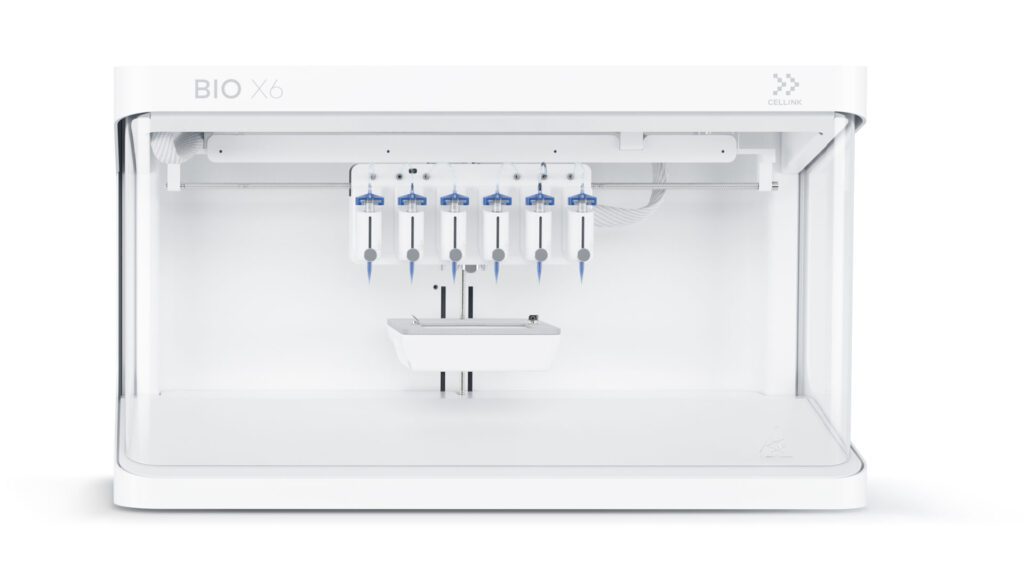
BIO X6
Coaxial printing, multiple printheads and material flexibility allow for greatly customizable microfluidic chips which can, for example, be used in conjunction with a patient’s own cells in a personalized medicine context.
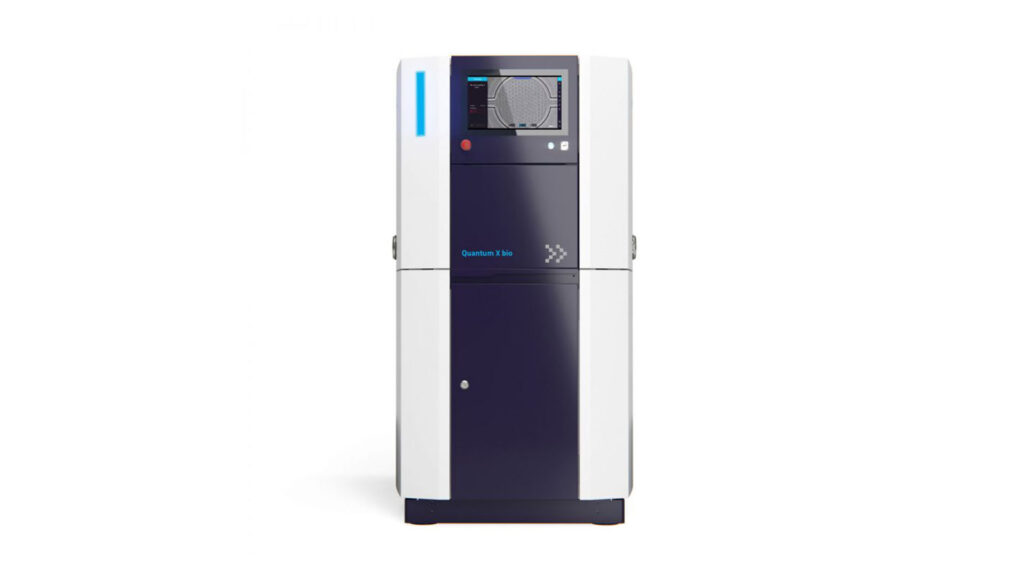
Quantum X bio
With the Quantum X bio, a powerful 3D microfabrication system, you have the flexibility to print on a variety of substrates: including microfluidic system for organ- or lab-on-a-chip applications.